My lab looks at how life experiences, aging, flight and pesticides affect learning, memory and brain structure in bees. I strive to involve undergraduates in my research whenever possible and two students are co-authors on my latest journal article, "Task specialization and odor effects on proboscis conditioning in Bumblebees" (J. Insect Behavior, 2013). Read on for a current overview of projects in “The Bee Lab”...
- A little background
- Project 1: Is foraging stress is correlated with cognitive performance and cellular markers of metabolic aging?
- Project 2: Do bees show age-related changes in learning performance and brain structure?
- Project 3: Can the bumblebee provide a non-mammalian model for pesticide induced Parkinson’s disease?
- REFERENCES
A little background
An animal’s brain can show tremendous plasticity over the course of the animal’s life. It is widely accepted that these brain changes are correlated with changes in cognitive function. For example, at birth the human brain is only 25% of its adult volume, but the brain grows rapidly; this growth is correlated with an extraordinary increase in cognitive ability. The increase in brain size and cognitive function does not involve a substantial addition of new nerve cells. Rather, neurons that were already present at birth form new and more complex synaptic connections, leading to an 8-fold increase in gray matter volume by age 6 (Matsuzawa et al, 2001). Severe stress is associated with both a failure to see the expansion of synapses and impaired cognitive and social skills (McCrory et al, 2010). Changes in brain morphology are also present at the other end of life -- during aging -- and include a decline in total brain weight and cortical thinning (loss of synapses). These changes are accelerated in neurodegenerative diseases such as Alzheimer’s disease, providing support for the idea that the number of synaptic connections is tightly linked to cognitive ability.
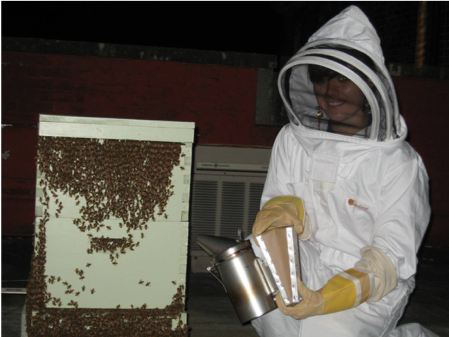
Research over the few decades has begun to reveal the biological mechanisms underlying brain aging and associated cognitive senescence. We now know that normal aging is not associated with or explained by a significant loss in neuron number. Instead, the biological basis for the declines in cognitive and memory functions appears to lie at the level of synaptic activity; thinning of the cortex and loss of brain volume are due to a decline in the number of synaptic contacts and/or in their efficacy of synaptic transmission (Toescu, 2005). Moreover, an increasing body of evidence suggests that, at least in mammals, brain aging is associated with mitochondrial dysfunction. This metabolic model of aging posits that as neurons age their mitochondria cease to function well, leading to less available ATP, a reduction in calcium buffering ability, and more free radical production. Together these changes leave the neurons more vulnerable to insults such as those that might result from traumatic brain injury (falls), disruptions in energy supplies (strokes), accumulation of toxic products (plaques in Alzheimer’s disease), or environmental toxins (Parkinson’s disease) (Salat et al, 2004; Toescu, 2005).
Scientists have exploited several animal models to study the effects of experience and chronological age on brain function. Among these model systems social insects, such as bees, provide a powerful tool (Jemielity and Keller, 2007). Bees live in social groups and show task specialization – meaning that different individuals in the colony take on different tasks, analogous to the heterogeneous experiences of humans. Some worker bumblebees spend their entire life as in-hive workers, feeding and incubating brood; other workers leave the hive to forage for nectar and pollen. I examine how chronological age, different behavioral experiences, and oxidative stress levels impact cognitive ability and synaptic volume in bumblebees.
Current Research Questions
Project 1: Is foraging stress is correlated with cognitive performance and cellular markers of metabolic aging?
Working with students, I have previously investigated whether worker bumblebees specializing on foraging versus in-hive tasks show differences in their ability to learn to associate an odor with a sugar reward. We found that while both foraging and in-hive specialists could learn the task, the percent of bees that learned was much higher (2x) among the in-hive specialists than among the foraging specialists (Hannaford et al, 2013). This result is surprising, because this behavioral paradigm is thought to model flower learning (in the normal realm of foragers) and also because some honeybee studies have shown that forager specialists show superior learning to in-hive workers. While there are several possible explanations for our results, one intriguing possibility is that the foraging bees are showing cognitive senescence due to damage sustained during metabolically-intensive foraging behavior. Foraging requires tremendous physical resources. Bees may fly for over a mile to find food, and flight is metabolically costly. Foraging honeybees have a metabolic rate of 100 to 120 mL O2g-1h-1, which is the highest mass-specific metabolic rate known and is 10 to100 times higher than in nurse bees (Suarez et al., 1996). Moreover foraging may exposes bees to environmental stressors such as UV light. Taken together these stressors may result in cellular level damage that leaves foraging specialists vulnerable and less adept at learning. Work in honeybees has shown declines in cognitive ability in aging foragers. In both an olfactory conditioning paradigm (Behrends et al., 2007) and tactile conditioning paradigm (Scheiner and Amdam, 2009) foragers with more than 15 days of experience are slower to learn than either foragers with only 1 week of foraging experience or in-hive specialists. We also know that neural oxidative damage in the brains of honeybees is more strongly linked to social role than to chronological age (Seehuus et al., 2006). Flight activity increases oxidative stress and mortality in houseflies and reduces oxidative stress resilience in fruit flies (Yan & Sohal, 2000). Additionally, intense exercise has been shown to increase mechanical deterioration of the wings (Finch, 1990), lower flight capacity (Vance et al., 2009), and reduce frequency of foraging trips (Tolfilski, 2000).
We can set up bumblebees to forage in three conditions and then assess their ability to learn.
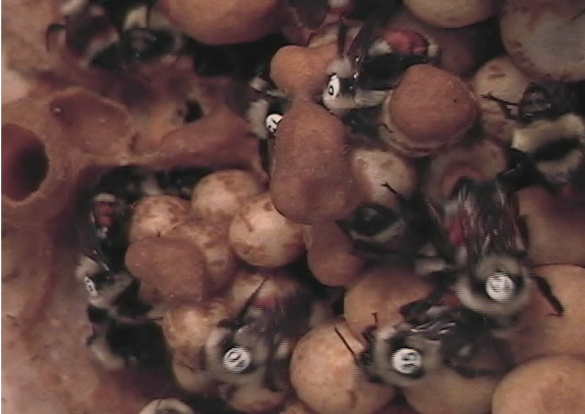
The three foraging conditions are: closed colonies (no flight behavior, only walking), flight-cage colonies (foragers will have to fly to distant artificial feeders with nectar), and free-foraging colonies (hives open to the outdoors, foraging in nature).
We can uniquely tag each bee so that we can quantify the behavior of each worker (image above). We videotape the hives to identify the time each bee exits and re-enters the hive, and we will note if the bee is carrying pollen or nectar back to the hive.
Then we pull bees from each of the conditions and assess their ability to learn using the well-characterized proboscis extension conditioning (PEC) assay (image below) (see Hannaford et al, 2013 for methods).
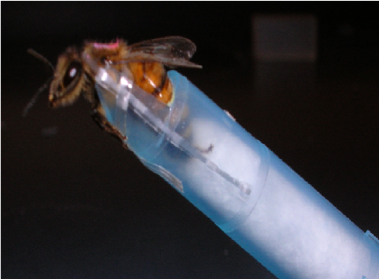
After the behavioral testing, we flash freeze the bees in liquid nitrogen. We then dissect out the brains and assess levels of oxidative stress on the bees.
There are several markers for oxidative stress. We have found that flight experience negatively impacts the levels of catalase (Figure 1, below).
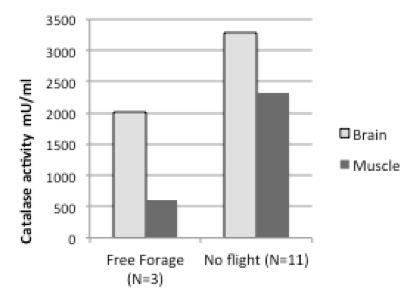
We found similar results for muscle tissue, consistent with previous studies on honeybee flight muscle (Williams et al., 2008). While the sample size in our pilot studies is small, the results show that it will be possible to quantify behavior in individual bees and see if antioxidant markers are correlated with performance in uniquely identified bees.
Project 2: Do bees show age-related changes in learning performance and brain structure?
I previously examined bumblebee brains to determine if age and the experience influenced brain morphology. We found a clear increase in the volume of Mushroom Bodies (MB), the brain region involved in learning and memory, over the first few days of adult life. Within the first 4 days of adult life, bumblebee MB synaptic volume increased by 20%. This work confirms other studies done in another social insect, the honeybee. The honeybee studies have also shown that, as in mammalian plasticity, the expansion in the forager honeybee MBs is not due to the addition of new neurons, but rather to an increase in the volume of synapses, and these changes are touted as being a model for the expansion in gray matter and cognitive skills seen in young mammals.
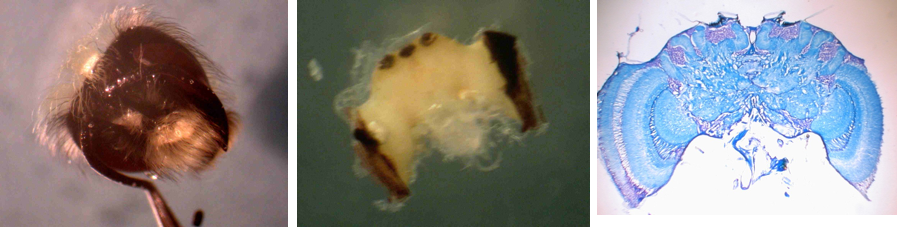
My earlier work also showed another intriguing finding. When we quantified the activity of individual bees, we found bees that are more active (higher foraging rate for foragers, higher in nest activity rate for the in-hive specialists) have larger synaptic volumes than less active nest-mates in similar “job.” The strong correlation between activity and brain structure provides additional support for the hypothesis that synaptic volume is positively correlated with the ability to carry out complex tasks.
Given the parallels between our results in bumblebees and those seen in mammalian systems, want to test the hypothesis that old bumblebees show cognitive senescence and that this decline in learning ability is correlated with a decrease in synaptic volume. I will address this question by raising bumblebees of known age, assessing their cognitive ability, and then quantifying the volume of their MBs.
As expected very young bees (1 day old) performed very poorly on a learning task, while nearly all bees between 1 and 3 weeks of age (the age at which they would normally be foraging) were able to learn the task. Intriguingly, very old bees (5-7 weeks of age) did not perform as well as younger bees, suggesting that bees show cognitive senescence (Figure 2, below).
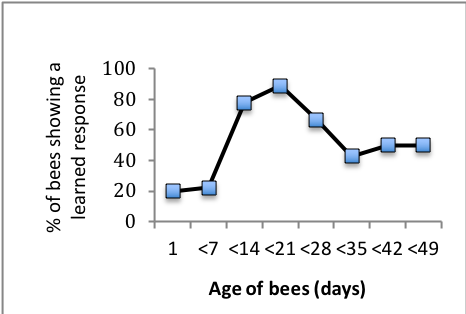
I want to expand this study. I expect that the largest synaptic volumes will be seen in “middle aged” bees, consistent with their learning performance. Among aged bees, I predict that those that fail to learn the PEC task will show a decline in the synaptic volume of the MB, and that the proportion of bees failing to learn will increase with increasing age. In addition, if increasing metabolic demands leads to accelerated aging, then the relative onset age for cognitive senescence should be earlier for bees in the flight cage condition, since those bees will need to fly to forage.
Project 3: Can the bumblebee provide a non-mammalian model for pesticide induced Parkinson’s disease?
Paraquat (N,N′-dimethyl-4–4′-bipiridinium) is one of the most commonly used herbicides worldwide¹. When inside a living organism, paraquat (PQ) strips electrons from the electron transport chain and is reduced to a free radical, which leads to the creation of a reactive oxygen species (ROS) called the superoxide radical (O2•–)3. The antioxidant enzymes superoxide dismutase (SOD) and catalase work together to rid the body of harmful O2•– ⁴. When antioxidant defenses fail, cells undergo apoptosis, which is marked by fragmented DNA and the activation of caspase enzymes. High levels of ROS are known to contribute to the development of neurodegenerative diseases including Parkinson’s disease (PD), Alzheimer’s disease, and amyotrophic lateral sclerosis (ALS). PD is characterized by reduced motor control, changes in speech, and memory loss in later stage.
Given that pollinators, such as bumblebees, may regularly exposed to pesticides as they forage, we have been asking if feeding bumblebees paraquat affects their movement, the antioxidant levels in their brains, and the levels of apoptosis in their brains. If paraquat consumption has an effect in bumblebees similar to that seen in mammals, they might serve as an alternative model for Parkinson’s disease.
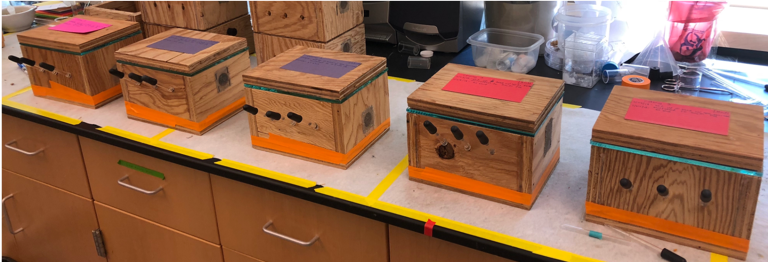
Behrends A, Schiner R, Baker N, Amdam G (2007) Cognitive aging is linked to social role in honey bees (Apis mellifera), Exp Gerontol, 42: 1146-1153.
Elekonich MM and Roberts SP (2005) Honey bees as a model for understanding mechanisms of life history transitions, Comp Biochem Physiol A, 141: 362-371.
Hannaford S, Sattler A, Siegel J., Foster RL (2013) Task specialization and odor effects on Proboscis Extension Conditioning in bumblebees (Bombus huntii), J. Insect Behavior, 26:762– 26:762–779
McCrory E, De Britto SA, Viding E (2010) Research review: the neurobiology and genetics of maltreatment and adversity, J Child Psychol Psychiatry, 51: 1079-1095.
Jemielity S, Keller L (2007) Aging: A young mind in old bees, Current Biology, 17: 294-295.
Roberts SP, Elekonich MM (2005) Muscle biochemistry and the ontogeny of flight capacity during behavioral development in the honey bee, Apis mellifera, J Exp Biol, 208: 4193-4198.
Matsuzawa J, Matsui M, Konishi T, Noguchi K, Gur R, Bilker W, Miyawaki T (2001) Age related volumetric changes of brain gray and white matter in healthy infants and children, Cerebral Cortex, 11: 335-342.
Salat EH, Buckner RI, Snyder AZ, Greve DN, Desikan RSR (2004) Thinning of the cerebral cortex in aging, Cerebral Cortex, 14: 721-730.
Scheiner R and Amdam G (2009) Impaired tactile learning is related to social role in honeybees, J Exp Biol, 212, 994-1002.
Suarez, R.K.; Lighton, J.R.B.; Joos, B.; Roberts, S.P.; Harrison, J.F. Energy metabolism, enzymatic flux capacities and metabolic flux rates in flying honeybees. Proc. Natl. Acad. Sci. USA 1996, 93, 12616-12620.
Toescu E (2005) Normal brain aging: models and mechanisms, Phil Trans R Soc B, 360: 2347-2354.
Vance JT, Williams JB, Elekonich MM, Roberts SP (2009) The effects of age and behavioral development on honey bee (Apis mellifera) flight performance, J Exp Biol, 212: 2604-2611.
Williams JB, Elekonich MM, Roberts SP (2008) Age and natural metabolically-intensive behavior affect oxidative stress and antioxidant mechanisms, Exp Gerontol, 43: 538-549.
Withers GS, Fahrbach SE, Robinson GE (1993) Selective neuroanatomical plasticity and division of labour in the honeybee, Nature, 364:238-240.
Yan, L. J. & Sohal, R. S. (2000). Prevention of flight activity prolongs the life span of the housefly, Musca domestica, and attenuates the age-associated oxidative damage to specific mitochondrial proteins. Free Radic. Biol. Med. 29, 1143-1150.